|
Newsletter September 2021 | Menu of Newsletters
(2016 Legacy Article) The tunable
microwave-frequency alternating current scanning tunneling microscope (ACSTM)
has opened the possibility of recording local spectra and local chemical
information on insulator surfaces, much like the conventional scanning
tunneling microscope (STM) has done for metals and
semiconductors..
Spectroscopy in the microwave frequency range enables
unrealizable measurements on conducting substrates, such as the rotational
spectroscopy of a single adsorbed molecule. Developed in the early 1990s by
Professor Paul Weiss, the nano-pioneering Director of the Weiss Group, a
nanotechnology research unit of UCLAs California NanoSystems Institute, the
ACSTMs single-molecule measurement techniques have illuminated unprecedented
details of chemical behavior, including observations of the motion of a single
molecule on a surface, and even the vibration of a single bond within a
molecule. Such measurements are critical to understanding entities ranging from
single atoms to the most complex protein assemblies.We use molecular design,
tailored syntheses, intermolecular interactions and selective chemistry to
direct molecules into desired positions to create nanostructures, to connect
functional molecules to the outside world, and to serve as test structures for
measuring single or bundled molecules, said David McMillan, Lead Technician at
the Weiss Group.
Critical to understanding these variations has been
developing the means to make tens- to hundreds-of-thousands of independent
single-molecule measurements in order to develop sufficiently significant
statistical distributions, while retaining the heterogeneity inherent in the
measurements.
Vibration isolation To achieve
these nano-level chemical and spectroscopic data sets, the ACSTM must be
positioned in an ultra-stable operating environment, one free of low-frequency
vibrations.The lab was using almost exclusively optical tables on pneumatic
isolation, said McMillan.One of our big problems has been space constraint. We
needed smaller pneumatic optical tables to fit. But as the air tables get
smaller, their vibration isolation performance diminishes.
Full article... |
FP-1 Floor
Platforms for SEMs, TEMs, STEMs and other heavy
Microscopes
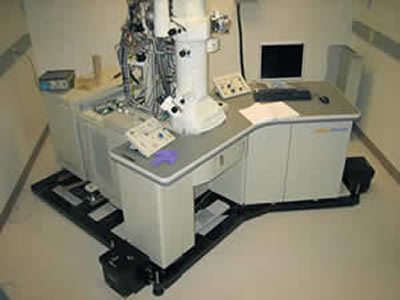
The
FP-1 consists of a
large platform, two to four Minus K®
SM-1
negative-stiffness vibration isolators, outriggers which connect the platform
to the isolators, isolator floor and jack screw plates which help to spread the
load on the floor and ballast weights. The
FP-1 platform is a
heavy steel plate which typically weighs nearly as much as the microscope
itself. One reason for this design is to lower the overall system center of
gravity to the level of the isolator tilt mechanism, so as to avoid
horizontal-tilt coupling, which occurs when horizontal vibrations cause both a
horizontal and tilting response of the column. With the system CG at the level
of the isolator tilt mechanism, the vibrations are decoupled and better
isolation control is achieved. Another reason for the massive platform is that
the large total mass of the system helps to diminish any effects from stiff
cables and hoses connected to the microscope.
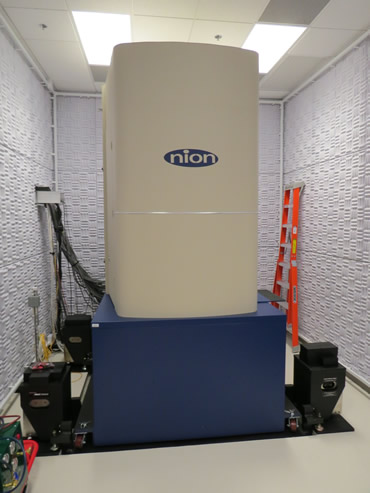
FP-1 Video:
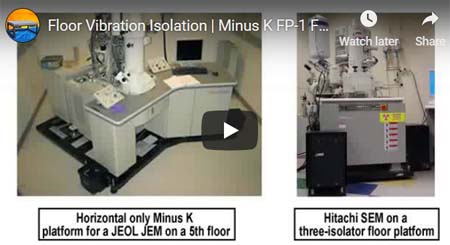
More images...
|
The compound microscope has evolved from an
instrument providing simple contrast viewing, into super-resolution systems
capable of sub-diffraction accuracy. Its two platforms, upright and inverted,
can be found in most laboratories doing cellular research, such as in biotech
and pharmaceutical. Upright microscopes are used for viewing glass slides, and
inverted for viewing live cells in Petri dishes. Despite its imaging advances,
the basic architecture of the compound microscope has not substantially been
modified in centuries.
This has now changed, with the recent release of
the Revolve hybrid compound microscope, developed by Echo Laboratories (Echo),
which has set a new precedent in microscope usability and design. The Revolve
combines the full functionality of both upright and inverted microscopes in one
instrument, and can switch between the two imaging modes relatively swiftly and
easily. This gives the flexibility to view many types of samples with one
microscope to a level of 300 350 nanometers resolution.
More than 70 percent of labs end up
having both inverted and upright microscopes, said Jeff Huber, Director
of Sales for Echo Laboratories. But both uprights and inverts use similar
objectives, illuminators, position systems and cameras. Why duplicate all of
these expensive components? And why take up valuable lab space with two
instruments? So, Echo Laboratories engineered a way to merge these two systems
into one unified instrument, which is the Revolve
microscope.
Brightfield, Phase Contrast and Epifluorescence
Imaging with iPad and Wireless Upload his is a compound, infinity-path
microscope, with applications for brightfield, phase contrast, and
epi-fluorescence imaging. Current glass selection includes the entire line of
Olympus objectives. Due to the unique nature of the upright/inverted
combination, both a high-NA and long-working-distance transmitted-light
condensers are available to support phase and brightfield. A high-accuracy
locking mechanism is used to securely hold the condenser assembly in place,
while still allowing for easy removal by a single lever.
Full article... |
Since the release of the first commercial
atomic force microscope (AFM) about 30 years ago, technology advances have
steadily been implemented to improve their performance. Now, the most recent
advance in ambient-temperature AFMs is making them more compact, portable and
user-friendly, which is enabled by Negative-Stiffness vibration isolation.
The atomic force microscope (AFM) has become one of the foremost tools
for imaging and measuring materials and cells on the nanoscale. Revealing
sample details at the atomic level, with resolution on the order of fractions
of a nanometer, the AFM is instrumental for imaging an array of applications,
such as defining surface characterizations, lithography, data storage, and
manipulation of atoms and nano-sized structures on a variety of
surfaces.
The AFM utilizes a sharp tip (probe) with a radius of
curvature on the order of a few nanometers attached to the end of a tiny
cantilever used to scan across a sample surface to image its topography and
material properties. When the tip is brought into proximity of a sample
surface, forces between the tip and the surface lead to a deflection of the
cantilever. This deflection is recorded using, typically, a laser beam that is
reflected from the top surface of the cantilever to a photo-sensitive detector.
The resultant change of position of the cantilever/probe/tip permits
characteristics such as mechanical, electrostatic, magnetic, chemical and other
forces to be precisely measured by the AFM. These characteristics are displayed
in a three-dimensional surface profile of the sample (in the X, Y and Z axes),
an advantage that the AFM can provide compared to other microscopy
techniques.
Although AFM technology has advanced considerably, its
benefits have not always been easily accessible for researchers requiring AFM
adaptability, portability. Nor have AFMs been adequately accessible in
nanotechnology student laboratories, because of lack of student skill in their
operation, and budget limitations on the number of AFMs at their
disposal.
A More Portable, More User-Friendly
AFM These inhibitions have now been mitigated by a relatively new
compact, portable and user-friendly ambient-temperature AFM. Developed by
NanoMagnetics Instruments, a leading manufacturer of scanning probe microscopes
for low-temperature applications, the ezAFM+ atomic force microscope is a
benchtop instrument designed for short learning times, quick setup, and ease of
transport.
Full article... |
|
|
|
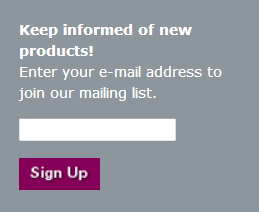
 |
|
|