Newsletter March 2025 | Menu of
Newsletters
The worlds only benchtop
transmission electron microscope (TEM), from Delong Instruments, combines
high-contrast imaging with nanoscale resolving power. The low-voltage electron
microscope can operate in four imaging modes: TEM; scanning EM (SEM); scanning
transmission EM (STEM); and electron diffraction; without having to realign the
column or adjust the sample, allowing both surface and transmission images of a
sample to be captured from the same area of interest. Negative-stiffness
vibration isolation enhances the performance of the instrument in
vibration-challenged noisy environments.
Transmission electron
microscopy (TEM) utilizes a technique in which a beam of electrons is
transmitted through an ultra-thin specimen, interacting with the specimen as it
passes through. An image is formed from the interaction of the electrons
transmitted through the specimen. The image is magnified and focused onto an
imaging device, such as a fluorescent screen, a layer of photographic film, or
a sensor such as a CCD camera. TEMs are capable of imaging at a significantly
higher magnification than light microscopes. This enables the investigators to
examine fine details, even as small as a single column of atoms. As the
wavelength of an electron can be up to 100,000 times smaller than that of
visible light, electron microscopes have a much higher resolution of
approximately 0.1 nm.
TEM is a powerful tool for directly looking at
small physical specimens. The microscope has evolved into a sophisticated
instrument capable of providing structural and chemical information from solid
materials over a wide range of magnification, to a level of spatial resolution
that is unapproachable by most other techniques. The TEM offers a wide variety
of imaging, diffraction, and microanalytical modes that can be used
individually or in combination to extract essential information. As long as the
instrument is aligned and used properly, many TEM techniques are available to
researchers to reveal a wealth of information about their samples.
A
recent development is the benchtop TEM, which enables a heightened level of
location flexibility and user- friendly applications for researchers engaged in
high-resolution microscopy.
Nanoscale from Your Benchtop The
worlds only benchtop TEM design, the LVEM5 electron microscope combines
high-resolution imaging with nanoscale resolving power (Figure 1). Designed and
manufactured by Delong Instruments (Delong), the low-voltage electron
microscope has the ability to operate in four imaging modes: TEM; scanning
electron microscopy (SEM); scanning transmission electron microscopy (STEM);
and electron diffraction (ED); and it provides data wit out having to realign
the column or adjust the sample when changing operating modes. This allows both
surface and transmission images of the sample to be captured from the same area
of interest.
The LVEM5 is designed to produce detailed and meaningful
image results with unmatched contrast of biologic and soft mate- rial samples
and is a benefit to any lab working with, researching, or producing nano
materials. The LVEM5 is approximately 90 percent smaller than classical EMs and
is small enough to fit anywhere nanoscale imaging is needed. It is easy to
learn, operate, and maintain, at a fraction of the cost of a conventional
TEM.
Sensitivity to Vibration in Noisy Environments TEM, like
other high-resolution microscopy, is influenced by factors such as magnetic
fields, barometric pressure changes, room temperature variations, grounding
problems, and floor vibrations. Floor vibrations can originate from movement of
outside vehicle traffic, elevators, HVAC systems, building pumps and motors,
and ancillary equipment providing support for the microscope. Both vertical and
horizontal vibration can negatively influence the focus and resolution of the
images being viewed. The benchtop LVEM5 is designed to be well-isolated from
outside fields and vibrations, providing an adequate level of stability for the
instruments installed in the average lab environments. However, these
high-resolution instruments are most sensitive to low-frequency vibration, in
the range of a few Hertz (Hz), which are prevalent in noisy environments. These
low-Hz vibrations are challenging to eliminate from the microscopes
environment...
In combination, the LVEM5 and
negative-stiffness
vibration isolation systems provide a versatile system for
electron microscopy imaging in many environments for several types of samples.
The small footprint of the system provides an ideal instrument for limited
space environments.
Full
article... |
|
| | | |
|
Compact CM-1
Low Frequency Vibration Isolator
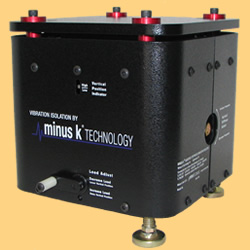 |
The
CM-1
is a compact
high capacity, low-frequency negative-stiffness isolator. As with all Minus K
isolators, they are completely passive and use no air or electricity. The
isolators can be combined into multi isolator systems to support heavy payloads
while taking up very little room themselves.
- Dimensions: 7.875" W x 7.875" D x 8.5" H (200mm W x
200mm D x 216mm H)
- Vertical natural frequency of 1/2 Hz or less can be
achieved over the entire load range.
- Horizontal natural frequency is load dependent. 1/2 Hz
or less can be achieved at or near the nominal load.
|
Get better
isolation than your air table KEEP YOUR your table top and replace the
air legs with our CM-1 isolators. No need for air compressors, air lines or
electricity
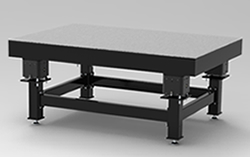
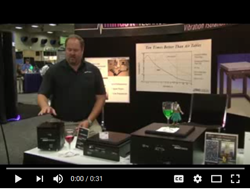 CM-1
Video
|
Pricing
& sizes for CM-1
Specifications
(pdf)
| |
Astronomers have confirmed more
than 5,000 exoplanets orbiting distant stars. There are a few aspects that are
needed to characterize an exoplanet. One is the size of the planet, and the
other is the mass of the planet.
Space-based missions, such as the
Transiting Exoplanet Survey Satellite (TESS) launched in 2018, find exoplanets
through events called transits, where a planet periodically blocks part of the
light from its host star. These space-based missions do the precision
photometry that is used to derive both the size of the planet - how big it is
physically, the planet's diameter - and how far away from the star it is. TESS
finds the most promising exoplanets orbiting our nearest and brightest stars,
Earth-sized and super-Earth-sized exoplanets which are no larger than twice the
size of Earth.
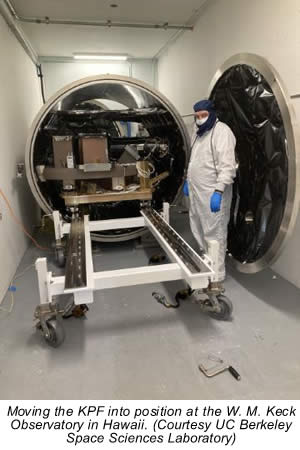 |
Once
space-based observations of an exoplanet are made, follow-up ground-based
measurements are needed to characterize the mass of the planet. This is
achieved by determining how fast the planets star is moving, and its radial
velocity, and needs to be performed with extreme precision, which to date has
only been demonstrated from a ground-based location. Once measurements of the
size and the mass of the planet are known, its density can be determined, which
enables an assessment of the planets composition - whether it is a gas giant or
a small rocky planet, or something in between.
Keck Planet
Finder Ground-based radial velocity (RV) measurements are made from
dozens of observatories around the world. Many are located at smaller
facilities where routine observation is possible. To reach the precision to
characterize earth-like planets, RV measurements need to be made from large
telescopes at exquisite observing sites. |
Until recently, many
ground-based radial velocity measurements were performed with the
High-Resolution Echelle Spectrometer (HIRES), located at the W.M. Keck
Observatory (WKMO) on the summit of 13,796-ft Mauna Kea in Hawaii. HIRESs
precision measurements can spot stars moving back and forth or wobbling, at a
speed of about 200 cm/second.
But
scientists wanted to push the technology further to permit locating even
smaller planets that exert a weaker tug on their host stars. A planets gravity
causes this solar wobbling, very slightly affecting the movement of the star.
More massive planets with higher gravity are easier to detect than smaller
rocky planets.
This led to the development of the Keck Planet Finder
(KPF), a next-generation optical spectrometer, also located at the W. M. Keck
Observatory in Hawaii, that saw first light in November 2022. Like HIRES, KPF
was designed to discover and characterize extrasolar planets using the radial
velocity method, but with much higher precision, capable of detecting stellar
motions of only 30 cm/second
First envisioned in 2014, KPF has been
jointly designed and built by the University of California, Berkeley Space
Sciences Laboratory (SSL) in Berkeley, California, and the California Institute
of Technology in Pasadena, California.
|
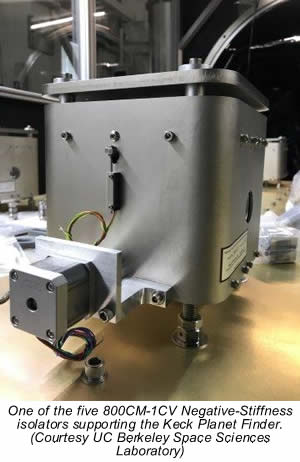
|
Since its
inception in 1959, SSL has participated in over 50 NASA space science missions,
including the Apollo, Mars, Discovery, and Explorer programs, as well as many
international space missions, said Kodi Rider, project manager of Keck Planet
Finder at UC Berkeley Space Sciences Lab. KPF is focused to not only find
smaller rocky planets, but ones in the habitable zones of their stars. Its
precision enables unprecedented measurements of the masses, orbits, and
compositions of smaller planets.
Thermal Stability KPF was
designed from the ground up to track the spectral fingerprints of stars to
better than one part-per-billion precision. This scale of measurement
represents a significant technological challenge and required every layer of
the KPF system to be optimized to maximize performance...
Full
article... |
|
| | | |
|
Previous
Newsletters
|
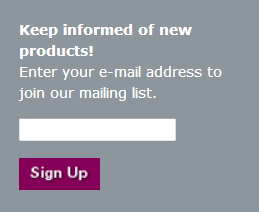
 |
|
|